Some factors adversely affect the efficiency of anionic flotation of phosphate in the absence of sodium silicate, thereby decreasing the concentrate grade. Impurities originally present on the quartz surface might be responsible for this adverse effect, but the precipitates heterocoagulated onto the surface of quartz also appeared to play a role. Dissolved ions, especially Ca++, can be present in considerable amounts in plant water leading to CaCO3 precipitation when the pulp pH is raised to 9.4 with the addition of ammonia.
Sodium silicate is used in the mineral industry ranging from the dispersion of siliceous gangue and slimes, in selective flocculation, mining and refining of clays, to the depression of gangue minerals in ore flotation. The structure of sodium silicate with Na2O/SiO2 ratios ranging from 1.16:1 to 7.74:1 was studied by Lentz (1964). Sodium silicate was classified by the contents of SiO4, Si2O7, Si3O10 (SiO3)4 and poly silicate structures. Sodium silicate solutions contain monomelic silicate ions, polysilicate ions and polymeric silicate micelles.
Krishnan and Iwasaki (1982) reported a model on how calcium silicate precipitates affect the surface properties of quartz in iron ores. When Ca++ is mixed with sodium silicate, calcium and silicate ions interact strongly resulting in the formation of calcium silicate precipitates. The main dispersive action of sodium silicate on quartz is produced by electrostatic repulsion due to calcium silicate precipitates formed on the surface of quartz. An increased addition of sodium silicate removed calcium silicate precipitates from the surface of quartz. As the zeta potential of calcium silicate precipitates and quartz become more negative, strong electrostatic repulsion leads to the detachment of calcium silicate precipitates from the quartz surface.
In the present investigation, the dispersive action of sodium silicate is indicated in the photomicrograph of Figure 4(d), which shows that the quartz surfaces are virtually free of precipitates. Apparently, sodium silicate removed impurity minerals (mainly wavellite and carbonates) and calcium-bearing precipitates from quartz surfaces by dispersive action.
Flotation Kinetics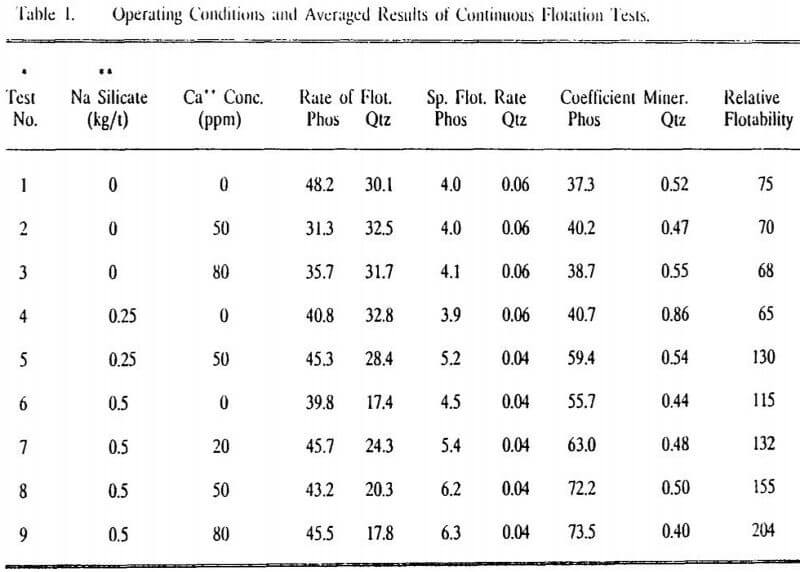
A study of flotation kinetics quantifies the flotation rates of minerals thereby providing information useful not only in the design and operation of flotation cells as well as circuits, but also in the evaluation of flotation reagents and their schedule. A kinetic model, proposed by Schuhmann (1942) for continuous flotation tests, is the most straightforward and practical of the continuous flotation models and best applies to the present investigation with regard to the evaluation of froth characteristics. He quantified the rates of flotation using continuous flotation data by defining two kinetic parameters: the specific flotation rate and the coefficient of mineralization. The specific flotation rate is defined as the rate of flotation of phosphate per unit weight of the phosphate in the pulp. The coefficient of mineralization is defined as the ratio of the concentration of phosphate in the froth product to its concentration in the pulp. The specific flotation rate can be expressed as:
Q = R/C1·V……………………………………………………………….(1)
or
Q = Pc·Pa·F……………………………………………………………….(2)
where, Q is the specific flotation rate of a given pulp constituent; R is the rate of flotation; C, is the concentration in the tailing stream which is assumed to be equal to the concentration in the pulp body; V is the volume of pulp in the cell; Pc and Pa are the probabilities of collision and adhesion, respectively, between a particle and a gas bubble; and F is the froth stability factor.
The coefficient of mineralization, M, can be expressed as :
M = Cfroth/Cpulp………………………………………………………………..(3)
where Cfroth and Cpulp, are, respectively, the concentrations of a particular mineral constituent in the froth product and the pulp body of the cell. The coefficient is regarded as a measure of the effectiveness of the flotation operation in dewatering the pulp.
The effects of sodium silicate in the presence of Ca++ were studied by means of batch and continuous flotation tests, frothability tests. SEM observations and turbidity measurements. The following conclusions may be drawn:
- The surface contamination of phosphate ore had an important role to play in carrying the quartz over to froth products. Sodium silicate reacted with quartz particles and depressed them after its addition (batch flotation tests).
- The use of sodium silicate accelerated the flotation of phosphate relative to quartz, and also made the froth drier (continuous flotation).
- Calcium silicate precipitates served as a protective coating on bubbles and stabilized the froth, due to an increase in surface viscosity. These bubbles were very resistant to coalesce because the calcium silicate precipitates created a stable froth layer (frothability tests).
- Quartz particles in the concentrate were covered with some impurity minerals and chemical precipitates, which made the quartz particles floatable with the collector used. Quartz surfaces in the tailing products were virtually free of precipitates (SEM observations).
- The beneficial effects of sodium silicate were attributed to: (1) the removal of impurity minerals (mainly wavellite and carbonates) and calcium- bearing precipitates from quartz surfaces by dispersing them, (2) drier and more persistent froths stabilized by oily droplets containing calcium silicate precipitates, and (3) higher specific flotation rates of phosphate and increased selectivity of separation. It is noteworthy that the batch flotation tests did not show the effect as clearly as the continuous flotation tests.