Table of Contents
In discussing the crystallization of a granitic magma it is sometimes assumed that there is a simple pro rata increase of the water content of the residual liquid as crystallization proceeds; i.e., it is assumed that, when the proportion of crystals amounts to one-half, the amount of water in the liquid is doubled, and when the crystals are nine-tenths, the water is increased tenfold. In making this assumption the fact that hydrous phases can and do occur in a granite is ignored. These hydrous phases are formed in part by reaction of the liquid with early crystals and the extent to which they are formed will depend upon the opportunity for reaction. In general it requires much more time for the formation of a certain amount of a crystalline phase by the method of reaction with the liquid than by the method of mere subtraction from the liquid, but if the rate of cooling is sufficiently slow, there may be ample time for the reaction type of equilibrium to be maintained or closely approached. Thus in the very slow crystallization of a granitic magma containing, say, 0.5 per cent water, it may be that after the proportion of water has increased to some 2 or 3 per cent the tendency to use up water in the reactional formation of hydrous phases keeps pace with the tendency to increase water in the residual liquid as a result of subtraction of anhydrous phases. In such a case the last vanishing residuum of liquid would contain only 2 or 3 per cent water. The crystalline product would thus be a granite containing 0.5 per cent water; in short, all of the water (and other hyperfusibles) that were originally contained in the magma. At no time would there have been any highly aqueous residual liquid. Some such picture is probably the true picture of the crystallization of a large, deep-seated mass of granitic magma.
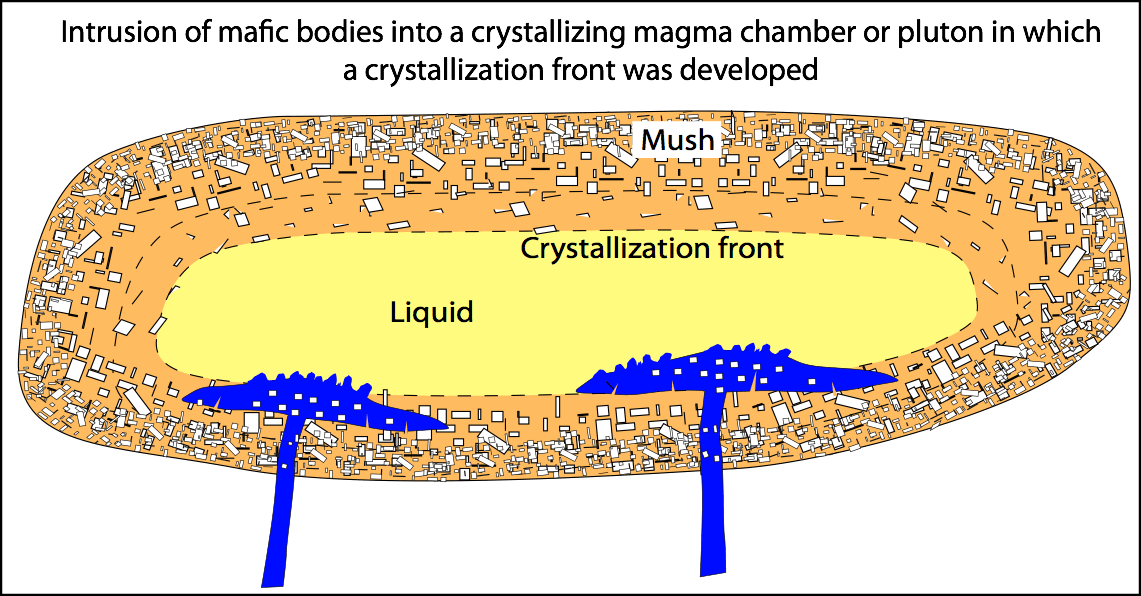
Under conditions permitting more rapid crystallization of the granite the amount of water in residual liquids will rise to greater values. A less deep-seated position of the magma will contribute to such behavior, and under such conditions a crystallizing granite may develop such a concentration of water in the residual, pegmatitic liquid that, in the light of Goranson’s results, boiling must be assumed to occur under the pressure there prevailing.
Goranson’s work shows that at 750° molten Stone Mountain granite can contain no more than 6.3 per cent water if the pressure is 980 bars, equivalent to a depth of some 3.5 km., and the pressure must be greatly increased in order to increase the solubility of water a relatively small amount. The solubility (at 900°) is increased less than 50 per cent by a threefold increase of pressure, and it would apparently require something like a tenfold increase to double the amount of water dissolved. While the addition of water to a melt made by fusing Stone Mountain granite does not precisely reproduce the conditions obtaining in the residual liquids of a crystallizing granite, nevertheless the approach is sufficiently close so that one may use the results as a guide. It seems inevitable that pegmatitic liquid sometimes boils even at rather considerable depths. Not only may boiling occur in the pegmatitic liquid that has occupied fissures, but often the portion that remains in the interstices of the parent mass may boil if the opportunity for its crystallization by reaction is inadequate. The boiling episode is of the utmost importance in connection with the formation of ore deposits, but before discussing this aspect of the matter it is necessary to consider the nature of the boiling liquid and of the vapor that is formed. Many of the hyperfusible constituents, but by no means all of them, can now be referred to as volatile constituents.
Fractional Distillation of Magmatic Residues
In the foregoing no little space has been devoted to discussion of the probability that residual liquids even of moderately deep-seated igneous masses frequently boil and that the vapor formed is of an acid character. It has also been pointed out that under less deep-seated conditions the vapors may escape at the surface and there deposit various materials. Nevertheless, in spite of these facts, it is not considered that a pneumatolytic process—i.e., deposition from the vapor phase—has any great importance in the formation of mineral deposits. The reason is that the history of the vapor is probably very different when it forms under deeper seated conditions. It is now necessary to direct attention to this question.
When residual magmatic liquid boils under deep-seated conditions it is an example of the so-called second boiling point. It is induced by the rise of vapor pressure of the liquid, consequent upon subtraction of crystals with falling temperature, and is thus contrasted with the ordinary boiling point, which is the result of rise of vapor pressure of the liquid, consequent upon rise of temperature. The boiling takes place at constant pressure equal to the external pressure (load). As boiling proceeds crystallization must also proceed. The number of components is large, the phases are few, so there can be no question of the system becoming invariant at the existing pressure. The temperature therefore continues to fall and the liquid continues to change in composition. The principal products of crystallization are silicates, and the liquid trend is toward an aqueous solution rich in chemical salts, though the extent to which the liquid will proceed in that direction cannot be predicted because the tendency to exhaustion of the liquid by reaction is ever present. In an actual example the vapor formed at any point will move only a short distance before it encounters a cooler environment where it is partly or wholly condensed, becoming a part of the liquid there existing. Though a full and accurate analysis of such a complex situation is not possible, it seems necessary to suppose that a mass of intrusive rock and its envelope, containing interstitially and in fractures a pegmatitic liquid that is experiencing “second boiling,” will constitute itself a fractional-distillation column. Throughout this column there occurs a liquid through which gas bubbles slowly rise and suffer selective (fractional) condensation. Close to the boiling source the liquid will be continually enriched in the hyperfusible constituents that are relatively non-volatile; at points more and more remote the liquid will become increasingly richer in those hyperfusible constituents that are more volatile—water, various halogen compounds including acids, and the other substances already suggested as prominent in the vapor phase.
Some light is thrown upon the change of composition of the liquid close to the boiling source by the observed changes in pegmatites, and much light is thrown upon the nature and activity of the acid solutions formed in the distillation column by the observed changes in the surrounding rocks (in part in the intrusive itself), for it is probably these acid solutions that are the principal agents of contact metamorphism, of vein formation, of replacement, and metasomatism in general.
The Acid Solutions and their Effects
It has been said that acid solutions formed by fractional distillation of pegmatitic residual liquors are probably the great agents of contact metamorphism, of vein formation, of replacement, and metasomatism in general. Many of the characteristic effects of these solutions probably could not be accomplished by gases (pneumatolytically) nor yet by alkaline solutions. It may indeed be questioned whether the residual liquids (alkaline) so long as they remain liquid will have any great tendency to escape from the igneous mass. It is possible that only when some vapor forms is there the necessary driving force for the escape of substances and for the diffusive penetration of the recondensed (acid) solutions.
The vapor that boils off from a crystallizing solution, say an ordinary salt solution, is in equilibrium with the liquid and with the crystals; in other words, it is saturated with the crystals and would not attack them in any way so long as it remained a vapor. But let the vapor be condensed at lower temperature and the liquid obtained will be wholly out of equilibrium (unsaturated) with the same crystals and will therefore attack and dissolve them. The same principle applies to the vapor given off from a crystallizing magma. It is saturated with all the crystals (silicates) of the magma, so long as it remains a vapor. Even upon crystallized silicates other than those formed from the magma it can have little power of direct solution. It could react with some of them to produce what may be termed addition compounds, hornblende from pyroxene, mica from hornblende, but the distinctive feature of many examples of mineralization is the outright solution and removal of minerals. When the vapor is condensed to a liquid, however, it can give precisely such effects. By the mere act of condensation to liquid, the formerly vaporous substances have been released from the bondage of their past, from the restrictions imposed upon them by their saturation-reaction method of derivation.
No important rock-forming mineral will be altogether immune to the action of these hot acid solutions, but there will be a wide range of susceptibility. We may perhaps form some estimate of the relative susceptibility of various minerals from the familiar facts of determinative mineralogy. One of the properties of any mineral ordinarily given in a textbook is its behavior toward acid, usually hydrochloric acid. Carbonates are, of course, readily attacked and taken into solution, and of the silicates it may be said that those loosely but conveniently termed basic are, in general, the more susceptible. It is therefore to be expected that carbonate rocks will be strongly attacked and, to a lesser extent, the igneous rocks containing plagioclase (labradorite to anorthite) and ferromagnesian minerals, especially if these have previously suffered some alteration to chlorite, serpentine, and epidote. On the other hand, rocks composed of alkaline feldspars and quartz will be less readily attacked. The clastic rocks will be susceptible to the extent to which their minerals correspond with those mentioned for the igneous rocks. The rocks most affected will therefore be carbonate rocks, the greenstones, the green schists, whether they are metamorphosed igneous rocks or metamorphosed impure slates, and, to some extent, the unmetamorphosed basic igneous rocks.
When the solutions attack these rocks the effects are, of course, mutual. The solutions are acid and of very complex character but among important constituents of their load are presumably sulphides and oxides of the heavy metals. The transport of sulphides of some metals in alkaline solution is a serious problem, as is also that of iron oxides. Their transport in acid solution is no problem at all. When the solutions act upon rock minerals they take materials into solution and deposit other materials and, since the taking of material into solution results in them more or less complete neutralization, among the substances deposited are those whose transport depends upon the solutions remaining acid. Sulphides and certain oxides are therefore among the substances deposited, and it is suggestive that the principal country rocks of ore deposits are the carbonate rocks, the greenstones and green schists, where they are invaded by igneous masses of acidic character, and to some extent the basic marginal phases of the igneous masses themselves.
We may examine somewhat more closely the action upon limestones. The principal effects are the removal of great quantities of lime and the introduction of many substances, notably iron and silica. Acid waters rich in chlorides are particularly suited to this activity. Lime is removed as chloride, and iron, introduced as chloride, is precipitated by lime as an oxygen compound, either oxide as such or in silicate combination. As a further effect, sulphides are deposited. Some question may be raised as to the ability of the initial acid solutions to transport silica, but these solutions, under pressure, carry, by hypothesis, SiF4 and SiCl4, or if one prefers, H2SiF6 and H2SiCl6.
It is characteristic of many limestone contacts that great masses of magnetite have been introduced and a corresponding volume of limestone removed. Gases could not remove the lime and it is very questionable whether alkaline solutions could introduce the iron.
In discussing certain historical aspects of the problems of ore deposition Lindgren states:
Intrusive masses were found to be normally accompanied by contact-metamorphic deposits which, in some cases, were connected by transitions with the swarms of veins that usually surround these igneous bodies as an aureole of metallic treasure. The great importance of this type for the solution of problems related to the genesis of ore deposits became clear to the minds of many investigators.
If contact-metamorphic deposits are in reality produced by the acid solutions formed in a fractional distillation column, then the swarms of veins connected by transitions with them must also be produced by the same solutions, though often after they have suffered much modification. In the attack of acid solutions upon silicates there is an ever-present source of free silica which, by analogy with the action of acids upon minerals in laboratory tests, we may assume to take, transiently at least, the form of gelatinous silica or even to remain temporarily in suspension (colloidal solution?) and to be susceptible of transportation. Is this the principal source of the almost universal quartz gangue of veins?
It may be objected that the solutions are being called upon to do too much and still remain acid, and the objection may be valid, but there is this to consider. It is probable that, in the passage of the acid solutions through the pores of rocks of the more susceptible types, any small element of the solution is quickly neutralized, but this small mass moves on and its place is taken by fresh solution. The system is necessarily a mobile one and on the average it may be that only at some distance from the intrusive source will all the solutions passing a given point be completely neutralized. At shorter distances the solutions may still be, on the average, somewhat acid. At greater distances the solutions will be alkaline, for this is the ultimate fate of hot waters in contact with silicate and other rock minerals. The fact that certain ore deposits are being formed at the surface through the agency of alkaline solutions is, therefore, no necessary barrier to the acceptance of the conclusion that most ore deposits are formed in depth through the agency of acid solutions. The alkaline solutions depositing cinnabar are, in this view, regenerated alkaline solutions.
There are many suggestions that the types of metasomatism characteristic of ore deposits formed at successive depths are systematically related to the changes in the solutions of the character noted. Not improbably a like connection is to be assigned to the tendency toward zoning of ores themselves with respect to their distance from the intrusive source. The writer has attempted to discuss these factors but the results are not very satisfactory and the matter is best left in the hands of those more familiar with the details of ore deposits. Some suggestions may be made very briefly.
There is a strong tendency toward a repetition of the igneous reaction series in the metasomatic products and gangue minerals of ore deposits. There is not, of course, complete correspondence of mineral species but with the highest temperature deposits iron-magnesium-calcium-rich minerals are the commoner associates, with the lowest temperature deposits alkaline feldspars, particularly adularia, the combined sericitic and chloritic types of metasomatism occupying, perhaps, an intermediate position. If the mineralizing solutions were commonly the alkaline residual solutions of magmas it is doubtful that such a relation would be found. The relations in the system are so complex that one cannot be dogmatic, but it does appear that such solutions would be supersaturated with cafemic (iron-magnesium-calcium) minerals and could only alter them. The facts are that cafemic minerals are not only altered but that with an adequate supply of solutions great quantities of such material are taken into solution, transported, and redeposited. Acid solutions formed in the manner pictured are capable of reacquiring practically all the constituent substances of even the more basic magmas and their deposition would be quite likely to reflect, in some measure, the reaction series of magmatic products.
As to the zonal relation of sulphides of the heavy metals in mineral deposits exhibiting increasing departure from the magmatic source, it will be said only that possibly the observed tendency is due to the order of their abundant precipitation from acid solutions suffering progressive neutralization.
Summary
The differentiation of igneous magmas follows a course basic to salic largely as a result of fractional crystallization. In the salic types there is, therefore, an enrichment of all substances of the original magmas that share with the salic ingredients the property of forming low-melting combinations and associations. Some of these substances have this property to a superlative degree and may be called hyperfusibles. They are principally water, sulphur, chlorine, fluorine, boron, and a number of others. As the salic magmas themselves crystallize, an increased concentration of the hyperfusibles in the residual liquid results. The trend is toward alkaline aqueous solutions, but with slow crystallization under heavy load the materials of these residues may be completely consumed in the formation of crystalline compounds that carry water, chlorine, boron, sulphur, fluorine, etc. Under less deep-seated conditions the residual liquid of crystallizing salic magmas frequently boils and a fractional-distillation column is set up in the interstices and fractures of the intrusive and its surroundings. This action results in the formation of a distillate which is an acid aqueous solution and it is such acid solutions that are probably the principal ore bringers. The solutions deposit their load as a result of reaction with the rocks through which they are forced as a consequence of the formation of vapor at the boiling source. They thus become neutral and finally alkaline, which is the eventual fate of hot waters in contact with rock minerals. Unless they are completely consumed in the formation of crystalline minerals (hydrous and the like) the regenerated alkaline solutions may approach the surface and, mingling with waters of the meteoric circulation, give rise to hot springs. In the less common case where the intrusive lies at very shallow depth, the distillate may reach the surface directly in the form of acid gases or occasionally acid waters.