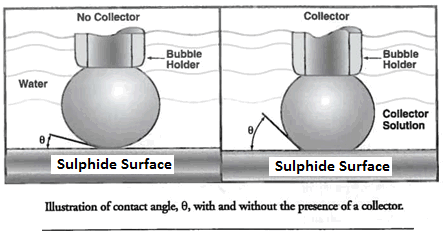
The separation of one component from another by flotation depends on the relative water-wetting ability of the surfaces of the various particle components. Many of the current chemical collectors in use are heteropolar, that is, they contain both a polar charged group and a non-polar uncharged group. When attached to a component surface, these surface active collector molecules are oriented so that the charged end of the molecule is attached or adsorbed to the component surface and the non-polar or hydrocarbon group is extended outward, thereby imparting a hydrophobic film to the particle. Typically, the surface free energy is lowered by the adsorption of heteropolar reagents so that the hydrophobic film can then act as a bridge allowing the particle to attach to a gas bubble.
The basic principles of surface chemistry have been applied extensively to the understanding of flotation phenomena. While some success has been achieved in fundamental studies, it generally is not yet possible to quantitatively predict industrial scale flotation performance very well from first principles. This problem is not surprising in light of the complex nature of flotation, involving complex chemical adsorption conditions in the presence of complex mass and momentum transfer conditions. Therefore, the practice of flotation is one that takes advantage of the qualitative guidelines offered by surface chemical studies coupled with actual flotation testing of reagents and other conditions in various sizes of flotation equipment A brief review of some of the surface phenomena that have shown useful correlations with flotation practice follows.
The first phenomenon of Flotation Fundamentals is the measurement of contact angle, 0, formed by an air bubble on a solid submerged in water. An air bubble, when put into contact with a clean surface, may or may not adhere to the surface. If the air bubble does not displace the aqueous phase on the parade surface, the contact angle is zero. Such would be the case for a highly hydrophilic material. However, if an appropriate chemical is added, the mineral acquires a hydrophobic film, or coating, and an air bubble may readily be attached. The presence of the collector significantly increases the contact angle, indicating enhanced particle-bubble contact. Typical materials demonstrating hydrophobicity will have contact angles between 45 and 90 degrees.
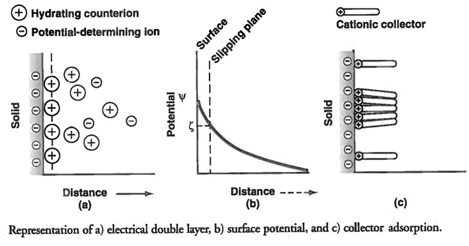
Another fundamental phenomenon useful in explaining flotation behaviour and selecting an appropriately charged collector is the concept of an electrical double layer. Component parades in aqueous solutions possess an electrical charge because of one or mote mechanisms, such as the existence of an excess of cations, or anions, at the solid surface due to solid defects, dissociation of surface groups in water, and unequal dissolution of lattice ions. On the left is a representation of the electrical double layer existing at a negatively charged particle surface. Attracted to this surface and extending into the solution are counterions (in this case, cations) that maintain electroneutrality. Potential-determining ions can be either part of the component structure or ions from the solution adsorbed onto the surface. The double layer extends into the bulk of the solution for a varying distance, depending on the valence and concentration of the counterions.
The potential measured by moving a particle through a solution is the potential at the slipping plane, denoted as zeta potential, The zeta potential is not the surface potential, but the potential at some distance from the surface. The sign of the charge on the surface, as measured by zeta potential, is very important, and the valence and concentration of potential- determining ions control the sign and magnitude of the surface potential. A point (or points) of zero charge, called the isoelectric point, can be measured for each material component, depending on conditions.
Measuring the contact angle. Place a drop of a liquid on a smooth surface of a solid. According to the wettability of the liquid in relationship to this solid, the drop will make a certain angle of contact with the solid. With reference to the figure 10, if the contact angle is lower than 90°, the solid is called wettable, if the contact angle is wider than 90°, the solid is named non-wettable. A contact angle equal to zero indicates complete wettability. To measure the contact angle use a protractor and a ruler. Taking a picture of the outline of the drop will make easier and more exact the measurement.
SURFACE PHENOMENA AND COLLOIDS
For example, for the material ceragyrite, AgCl, the potential-determining ions are Ag+ and Cl-. The AgCl material is positively charged in solutions containing greater than 10E-4 mole Ag+* per litre, requiring an anionic (negatively charged) collector. Conversely, the AgCl material is negatively charged in solutions containing Cl- in excess of 2E-6 mole per litre, requiring a cationic (positively charged) collector. The isoelectric point occurs at a pAg of 4.
For insoluble oxide materials, H+ and OH- are the potential-determining ions, so that the surfaces of these materials are positively charged at pH values below the isoelectric point and negatively charged at higher pH values. Polyvalent cations such as Al+3 and Fe+3 are also known to alter the surface charge of a material in aqueous solutions. This alteration is especially prevalent near the pH of Al+3 and Fe-3 ion precipitation in dilute solutions, causing specific adsorption of the precipitates on the material surface.
In recent years electrochemical techniques have been used successfully to explain the behaviour of sulfur-containing (thiol) collectors with sulphide minerals. This is a very important class of minerals because sulphide minerals are the source of most of the worlds base and precious metals. Briefly, experience has shown that in this particular type of flotation, the transformation of the sulphide mineral surface to a more hydrophobic state involves simultaneous electrochemical reactions in which the cathodic step, the reduction of oxygen, is coupled with an anodic step involving the oxidation of the collector.
It is becoming more evident that interfacial electrochemical potential is the governing factor in sulfide mineral systems using thiol collectors. At reducing potentials, the sulfide minerals are depressed, and with increasing oxidizing conditions, the same minerals are floated in the presence of thiol collectors. The location at which each sulfide mineral type will start to float as a function of electrochemical potential is relatively easy to determine in an idealized laboratory test using pure minerals and well controlled water chemistry conditions In a plant environment, this type of analysis is somewhat more difficult to predict, but nevertheless, the general concepts are still valid.
The detailed understanding of the mechanisms involved in Flotation Fundamentals for the attachment of collectors to various component materials, whether they be sulfide, oxide, or something else in composition, is far from complete at this time. The term adsorption is sometimes used to indicate a multitude of phenomena ranging from physical adsorption to chemisorption to chelation, depending on the particular system under study. In many industrial mixed-component systems, it is quite apparent that at least several simultaneous mechanisms are occurring with a single given collector. Attachment by chemisorption is generally desired because it leads to greater selectivity at lower dosage, hence at lower cost. Physical adsorption of the collector may involve the weaker forces of van der Waals hydrogen or hydrophobic bonding, with electrostatic attraction between an ionic collector and a mineral of opposite charge being the most common mechanism.
Currently, strictly chelation-oriented chemistries functioning as collectors are being evaluated for wide scale commercial use, but in practice their use at present is limited due to their higher cost. It is quite likely that many of the existing collector reagents such as the thiol collectors also exhibit what may be termed as chelation activity under certain conditions. In addition, some collectors undergo rearrangements at the component surfaces, leading to further complications in mechanism identification.
The differences in performance of flotation collectors in idealized systems (one component, pure water, air as a bubble former, etc.) compared to actual flotation systems (many components, highly impure water-even including seawater in some processes, oxygen enrichment of air, nitrogen, etc.) are great. Therefore, empirical testing in small (laboratory-scale) batch flotation cells, using hundreds of grams of solid mixtures as feeds followed by large scale plant verification, has played a significant role in collector chemistry development and use. The plant use of collector chemistry is extremely complicated and involves collector interactions with dosage, frother type, component particle size distribution, time rate of recovery, and so on. This topic will be discussed further in later sections of this module on Flotation Fundamentals.